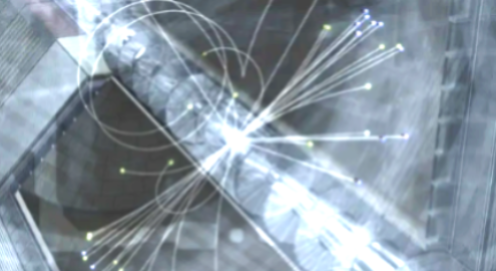
Positrons, the antiparticles of electrons, have a significant impact on various energy processes, from basic electricity to complex solar phenomena. Positrons have been studied and observed in numerous astrophysical contexts, including the Sun. This article explores the central role of positrons as the main mission-control in influencing and regulating energy production and conversion, highlighting their significant role from terrestrial applications to solar dynamics.
In solar process, positrons play a crucial role in the emission of solar gamma-rays, especially in the energy range from a few hundred keV to greater than 1 GeV. The Sun’s energetic environment, characterised by solar flares and coronal mass ejections (CMEs), creates the perfect setting for positron production and interaction. These solar eruptions involve processes such as magnetic reconnection, which accelerates particles to high energies, contributing to various emission mechanisms, including those involving positrons. The interaction of positrons with the solar environment leads to gamma-ray emissions. The most notable feature is the MeV annihilation line, which occurs when positrons excite electrons. This process can produce either a continuum of gamma-rays or discrete lines. Beyond their astrophysical significance, positrons have practical applications on Earth and beyond, such as powerful precision technique to screen and detect metabolic processes in living things, providing invaluable information for all types of diagnostics and monitoring purposes.
The Utmost Efficiency of Positrons
Positrons superbly exemplify control over energy through their unique interactions and the high-energy processes they facilitate. In the Sun, positrons are integral to the production of high-energy gamma-rays, influencing the overall energy regulation profile of solar directions. On Earth, positrons enable precision technologies, showcasing their utility in harnessing and manipulating energy at the quantum level, proving their dominance in both natural and technological realms. The annihilation of positrons with electrons is a fundamental process in high-energy astrophysics and laboratory physics, producing gamma-ray photons, typically at 0.511 MeV. The annihilation line profile during solar flare events can provide insights into the physical conditions and processes occurring in the solar atmosphere. Pair production is a pivotal process in high-energy astrophysics, involving the creation of particle-antiparticle pairs from a high-energy photon. The electron-positron pair is the most commonly discussed, due to the lower energy threshold compared to other particle pairs. The positron plays a dominant role in this pairing, and this importance cannot be replaced. Pair production requires the incoming photon to have energy exceeding the total rest mass energy of the created particles. In high-energy environments, such as near an atomic nucleus, a photon can convert its energy into an electron-positron pair. This process adheres to the conservation of energy and momentum, and typically requires the presence of a nearby nucleus to facilitate momentum conservation. The kinematics of pair production can be described using four-vector notation, ensuring conservation of energy and momentum. Neglecting the small recoil of the nucleus simplifies this relation, highlighting the nearly collinear emission of the electron-positron pair. This equation quantifies the kinetic energy shared between the electron and positron post-production. Positrons, especially in their pairing with electrons, are crucial in understanding high-energy phenomena in astrophysics and its impact on planetary settings. Their interactions provide valuable information on particle acceleration, transport, and interaction within the solar atmosphere. Spatially resolved gamma-ray observations are essential for further elucidating the roles of stochastic and shock accelerations in solar events
The Intrinsic bond of Electron and Positron: Rationale and Consequences of Separation
In high-energy physics, the electron-positron pair is a fundamental and inseparable duo. This pairing results from the intrinsic properties of particle-antiparticle relationships, governed by conservation laws and quantum mechanics. The positron plays a dominant role in this pairing due to its positive charge, opposite to the electron’s negative charge. This dominance manifests in various physical phenomena and interactions, including annihilation, excitement, entanglement and pair production. The inseparability of electrons and positrons is rooted in several fundamental conservation laws:
- Conservation of Energy: An electron (negative) is always paired with a positron (positive) to ensure the overall energy is conserved. Separating them would violate this principle.
- Conservation of Momentum: In pair production, a high-energy photon creates an electron-positron pair, converting energy into mass (E = mc²). Similarly, their annihilation returns energy back into photon form. These processes demand absolute presence of both electron and positron to maintain energy and momentum balance.
- Conservation of Lepton Number: Electrons and positrons belong to the lepton family, each with a lepton number of +1 and -1, respectively. Their pairing ensures the total lepton number remains zero. Separating them disrupts this balance.
- Astrophysical Implications: Gamma-Ray Bursts and Solar Flares: In high-energy environments, the balance of electrons and positrons affects the radiation emitted. Disrupting this balance would alter the observed spectra and energy distribution. Contrary to popular belief, it will further deprive energy of other already weak-energy particles.
Dire implications to the Electrons when separated
Electrons and positrons produced simultaneously are often quantum entangled. This means their properties are interdependent and non-transferable regardless of the distance between them. Separating entangled pairs alters the quantum state of the system, breaking the correlations and violating entanglement principles unless properly managed through quantum decoherence or measurement. Annihilation releases energy in the form of gamma rays. If electrons and positrons are separated beyond a certain threshold, they cannot annihilate efficiently, leading to an imbalance in expected energy distribution where particles such protons will be automatically deprived even more. The electron-positron pairing is fundamental in maintaining various conservation laws and ensuring the stability of physical systems. Separating these pairs leads to significant violation of fundamental principles and altering the behaviour of high-energy interactions that is hostile to weak-energy particles only. Consequently, the inseparability of electrons and positrons is crucial in both understanding and accurately modelling physical phenomena in astrophysics and laboratory settings. This elaboration highlights the importance of this unbreakable duo.
- Electrons: are more likely to encounter other particles or nuclei in most environments. These interactions can lead to various processes such as scattering, absorption, or radiation losses. In these conditions, the electron may lose energy quickly or be captured by positive ions, resulting in changes to its state or annihilation.
- Positrons: being rare, are less likely to encounter other positrons but more likely to encounter electrons. In environments with few free electrons or where magnetic and electric fields are structured to trap positrons, they can survive much longer. Additionally, certain materials and conditions can stabilise positrons, such as in positron traps used in laboratory settings.
The inseparability of electrons and positrons in high-energy environments is primarily due to their intrinsic similar properties but complementary feminine and masculine characteristic dynamics. Electrons, being more prone to interactions, do not survive independently for long. Positrons, due to their rarity and universal’s forces for stabilisation, can survive independently. This distinction highlights the unique roles and behaviours of these fundamental particles in different physical contexts. In environments where free electrons are sparse, positrons have a lower probability of encountering an electron quickly, thus increasing their survival time. In contrast, electrons are almost always in an environment with free electrons or nuclei, leading to immediate interactions and energy losses.
Separation Dynamics and Survival differences
The following highlights the different state of positron and electron each when they are separated:
Laboratory Conditions
- Positron Traps: Positron traps utilise electromagnetic fields to isolate and store positrons. These controlled environments allow positrons to exist independently, whereas free electrons in the same environment would quickly interact with other particles and lose their independence.
- Electron Storage: Storing free electrons in isolation is more challenging due to their higher likelihood of interaction with surrounding materials, leading to scattering or low-quality, messy recombination processes, responsible for violations of universal law.
Astrophysical Contexts
- Electron-Rich Environments: In stars and interstellar space, the density of free electrons is high, leading to rapid interactions and energy exchanges. Electrons do not survive for long in such environments due to continuous interactions and energy deprivation.
- Positron-Rich Environments: In certain astrophysical events, such as near pulsars or in certain types of radiation belts, positrons can exist independently due to structured magnetic fields and relatively lower densities of free electrons.
Quantum Mechanical Considerations
- Quantum States: Electrons in bound states (such as in atoms) are stable due to quantum mechanical principles. Free electrons, however, are prone to losing energy through radiation or interactions.
- Positrons, if isolated or in low-electron-density environments, can maintain their quantum state without the need for annihilation with non-lepton particles.
- Wave-Particle Duality: Both electrons and positrons exhibit wave-particle duality, affecting how they interact with their environment. However, due to the desperate need for interactions and abundant quantity, electrons when not paired with their natural partner positrons will suffer quicker loss of independent states.
Electron-Dominated Environments
- High Electron Density: In environments with a high density of free electrons, such as in metallic conductors or plasma states, electrons frequently interact with each other and with atomic nuclei. Despite these interactions, the fundamental stability of the electron is not compromised, but its energy state will be weaker.
- Magnetic and Electric Fields: Electrons can be superficially influenced by external fields, causing them to move or change trajectories. In strong magnetic fields, electrons can spiral along field lines, making them to have more will in finding and pairing with their natural partners, positrons.
Positron-Dominated Environments
- Low Electron Density: In environments with few or abundant free electrons, positrons can always survive. Examples include specially designed positron traps in laboratory settings where positrons are confined using electromagnetic fields but proven to prevail every time.
- Interstellar Space: In regions of space with low particle densities, positrons can exist for extended periods, even until and after they encounter an electron.
Positron’s role in High-energy creation
In terms of energy creation, the behaviour and potential of electrons and positrons differ significantly due to their distinct roles and interactions. Here’s a detailed look at their characteristics and capabilities:
Electrons
- Interaction with Matter: Electrons are fundamental particles that participate in a wide range of interactions with matter. In these interactions, they can transfer energy and facilitate chemical reactions, but without positrons, they do not typically produce high-energy outputs.
- Energy States: In atoms, electrons occupy specific energy levels, and transitions between these levels can release or absorb photons (light). However, these energy changes are usually in the range of a few electron volts (eV), which is relatively low compared to the energy scales involved in positron interactions.
- Promiscuity: Electrons are indeed highly interactive, participating in electrical conduction, chemical bonding, and thermal conductivity. While these interactions are crucial for many physical and chemical processes, they do not typically result in the creation of significant amounts of high-energy radiation or particles.
Positrons
- Annihilation with Electrons: When a positron encounters an electron, they annihilate each other, producing a pair of high-energy gamma-ray photons. This process releases a substantial amount of energy, equivalent to the rest mass energy of the electron and positron (each having a rest mass energy of 511 keV, totalling 1.022 MeV).
- Energy Production: Positron interactions with their natural partner, electron, are highly energetic and can produce gamma rays, which are high-energy photons. This makes positrons valuable in contexts where high-energy radiation is useful or required.
Comparative Analysis
- Energy Creation: Positrons, through pairing physically and naturally with electrons, are capable of creating high-energy photons, which are useful in various scientific and technological pursue and applications. This process is a direct and efficient conversion of mass into energy.
- Electrons’ Role: While electrons are essential for a multitude of processes, including electricity generation, chemical reactions, and thermal conduction, they do not inherently produce high-energy outputs without interacting with genuine positrons as the highest-energy in the rank and most powerful particles. Their role in energy creation is more indirect and often involves facilitating or mediating processes rather than being the primary source of high-energy production.
What it means
Positrons as Powerhouses: Positrons can be seen as powerful sources of energy especially when they encounter electrons, leading to high-energy gamma-ray production. This makes them highly valuable in scenarios requiring significant energy output.
Electrons as Versatile but Low-Energy: Electrons are versatile and essential for numerous everyday processes, but on their own, they do not generate substantial energy. They are more involved in supporting roles, enabling various processes without being the primary source of high-energy production.
These can be easily translated into: positrons have the unique capability of producing high-energy gamma rays through pairing with electrons, making them significant contributors to high-energy phenomena. Electrons, while indispensable in numerous physical and chemical processes, do not independently produce high-energy outputs. Their interactions and contributions are crucial for the functioning of many systems but are not typically characterised by the creation of high-energy radiation or particles. In essence, a smart and clever electron seeking to maximise its impact and avoid a mundane, low-energy existence bound for elimination should strive to pair with a positron. This partnership transforms the electron into a source of high-energy radiation, contributing significantly to medical, technological, and scientific advancements. Thus, the electron’s ultimate value and longevity are best realised through its natural and physical interactions with a positron, ensuring it plays a crucial role in some of the most energetic and valuable processes beyond the unknown. What if an electron already in below-par pairing (with non-positron particles) encounter a positron and intend to create high-energy interaction? As the smartest and most powerful antimatter, the positron will ensure such energy output can never be shared with those deemed them competition or enemy.
Dependence of Electrons on Positrons. Positron Dominance in Energy Creation
- High-Energy Annihilation: The annihilation of an electron with a positron is a unique and powerful interaction that releases a considerable amount of energy. This process is critical for applications that require high-energy outputs, such as in medical imaging with positron emission tomography (PET). In this context, the electron’s role is almost passive, merely serving as the counterpart to the dominant positron, which drives the energy release.
- Lack of Independent High-Energy Production: Electrons, on their own, cannot produce high-energy photons or significant energy outputs. Their role in energy production is typically indirect, involving mediating or facilitating processes rather than being the primary source of energy. This dependence on positrons highlights the electron’s reliance on its antiparticle for generating substantial energy.
Electron’s Stability and Versatility
- Stable Pairings with Matter: Electrons are highly stable when paired with other matter, forming atoms and molecules. They are integral to chemical reactions, electrical conduction, and thermal properties. However, these stable pairings are merely low-energy processes, and the electrons’ interactions in these contexts do not result in the production of high-energy particles or prolong their existence.
- Replaceability and Vulnerability: In many physical and chemical systems, electrons can be easily replaced or even eliminated without significantly disrupting the overall system. For instance, in metal conduction, an individual electron is one of many contributing to the overall current, and its removal or replacement does not significantly impact the system. This replaceability underscores their secondary role compared to the transformative power of positrons in energy creation.
Electrons, while fundamental to countless processes in nature and technology, rely heavily on positrons for high-energy interactions and significant energy production. Positrons, with their ability to annihilate electrons and release substantial energy, are the dominant players in this relationship. Electrons can exist and function in stable pairings with matter, but without physically pairing with positrons, they lack the capacity for substantial energy creation. This dependence underscores the unique and powerful role of positrons in the realm of high-energy physics and applications, positioning electrons as versatile but ultimately secondary in high-energy contexts.
Final Thoughts
When a positron meets its matter counterpart, an electron, they annihilate each other, releasing gamma-ray photons with high energy. This phenomenon holds immense theoretical potential for future spacecraft propulsion and energy generation due to its efficiency and high energy release per unit mass. Although currently impractical due to technological and cost constraints, antimatter has been speculated as a potential future energy source for space travel and galaxy exploration. The efficient conversion of matter to energy in annihilation processes suggests a high energy density that could revolutionise energy production if harnessed on a large scale. In contrast to positrons, which possess direct and efficient energy conversion through annihilation, other particles like protons and neutrons are comparatively less relevant in high-energy equation. While protons and neutrons can be useful for nuclear stability and various energy production methods today, their roles are more constrained thus irreplaceable compared to the transformative energy potential of positrons. Positrons’ unique ability to release energy at nearly 100% efficiency through annihilation with electrons positions them as the forefront of future energy technology, relegating other particle-based energy processes to secondary roles. This transformative capability underscores the profound impact positrons have across diverse fields, ensuring their central role in advancing high-energy physics, space exploration, medical diagnostics, and beyond. Therefore, as positron-based technologies continue to advance, their supremacy in energy creation and control is expected to further-diminish the relevance of other particle-based energy sources.
The positron stands as the epitome of high-energy prowess, commanding respect across scientific, industrial, and strategic domains. Its unique ability to unleash vast quantities of energy through annihilation makes it indispensable in cutting-edge research, space exploration, military advancements, and the quest for sustainable energy solutions. As the world acknowledged the power of positrons, it becomes increasingly clear that their potential is limitless yet demanding. The challenges of energy-efficient production and utilisation are substantial but minute in comparison to the promise they hold for revolutionising energy generation, propulsion systems, and advanced materials science. In the realms of science and technology, the positron reigns supreme, driving innovation and pushing the boundaries of what is possible. As we navigate the complexities of unleashing its power responsibly, one thing remains certain: the positron is not just an important player of the future, it is the decider upon which groundbreaking discoveries and advancements will continue to be discovered. Therefore, in the pursuit of unlocking its full potential, one must only proceed based on the ideals and highest standard, for the positron is truly the king of energies, and its dominion should be approached with the utmost respect and ethics.