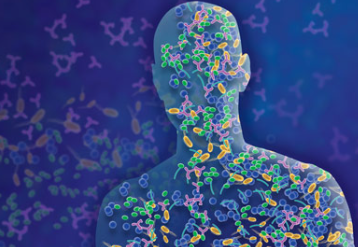
Human biology is experiencing a profound revolution, primarily influenced by advancements in genetics, medicine, and biotechnology. Alongside these developments, digitalization and technology have become critical tools, enabling the screening, detection, and identification of the origins, influences, and pathways of genome remodelling. These technological advancements are transforming our understanding of evolution and its connections to other areas of biology. Historically, between the late 19th and early 20th centuries, microbiologists gave considerable attention to the natural evolutionary relationships among bacteria, akin to the focus zoologists and botanists placed on metazoan genealogies.
Bacteria and Genome Remodelling
Bacteria are among the most prevalent living organisms, known for their ability to thrive in virtually all environments. Their adaptability is due to rapid evolution driven by their short generation times, large population sizes, spontaneous mutation rates, and genetic exchange across taxa. This adaptability allows bacteria to mutate and become immune to existing protective agents. These mutations and genomic modifications lead to new bacterial phenotypes that better suit their environments, a crucial aspect of bacterial evolution.
There are two principal evolutionary theories relevant to bacteria:
Post-Lamarckism: Proposes that external environmental cues lead individuals to change their phenotypes in response. Successful adaptations can be passed on to future generations.
Post-Darwinism: Suggests that evolution operates at the population level, where random genetic variations occur, and natural selection favours phenotypes suited to specific environments.
Fungi and Their Role in Ecology
Fungi are microscopic organisms that may be unicellular or multicellular, containing cellulose in their cell walls. They reproduce through sexual or asexual means and are classified as eukaryotic (with a membrane-bound nucleus) or prokaryotic (lacking internal membranes). As heterotrophs, fungi cannot produce their own food and derive nutrients from other organic sources, primarily plant or animal matter.
Fungi occupy various ecological niches and have evolved mechanisms to prevent the diffusion of harmful substances or pathogens. Notably, entomopathogenic fungi, which can eliminate insect pests, are being considered for use as biological control agents. However, their interactions with hosts and environments are diverse, ranging from mutualism to parasitism.
Of the estimated 5 million fungal species, a few hundred are parasitic and pose significant health risks to humans, particularly if they infect the nervous system. These infections can result in conditions like brain fog and delusional infestations, often affecting the skin.
The Role of Toxoplasma gondii
Among emerging infectious diseases, the zoonotic protozoan parasite Toxoplasma gondii is one of the most pervasive. It has been found in various mammalian and avian species, including humans. T. gondii is estimated to chronically infect one-third of the world’s human population, causing conditions such as ocular toxoplasmosis in otherwise healthy individuals and potentially fatal encephalitis in immunocompromised hosts. Vertical transmission from mother to fetus can result in severe birth defects.
The genetic diversity and virulence of T. gondii may have been influenced by human agricultural practices over the past 11,000 years. The domestication of cats and mice likely played a significant role in the parasite’s transmission cycle, shaping its evolutionary trajectory. Microbiology plays a critical role in influencing the evolution of species by impacting genetic diversity, ecological interactions, and adaptation processes. Microorganisms, such as bacteria, fungi, viruses, and protozoa, are involved in numerous interactions with other species, including symbiotic relationships, genetic exchanges, and environmental modifications. These interactions can drive evolutionary changes at both micro (genetic) and macro (species-wide) levels.
Here’s a look at how microbiology influences the evolution of species:
Horizontal Gene Transfer (HGT) and Genetic Diversity
Horizontal gene transfer (HGT) is the process by which an organism incorporates genetic material from another organism without being its offspring. Unlike vertical gene transfer (inheritance from parent to offspring), HGT can occur between different species, significantly contributing to genetic diversity and evolution.
Bacteria and HGT: Bacteria are known to exchange genetic material via mechanisms like transformation (uptake of naked DNA from the environment), transduction (DNA transfer by viruses), and conjugation (direct transfer of DNA between bacteria through cell-to-cell contact). These processes enable bacteria to rapidly acquire new traits, such as antibiotic resistance or the ability to metabolize new substrates, which can be spread across microbial communities and even to other species.
Impact on Evolution: The genetic material transferred can include genes that confer advantageous traits, such as resistance to environmental stressors, enhanced metabolic capabilities, or virulence factors. This genetic exchange contributes to rapid adaptation and can lead to the evolution of new species or the emergence of highly resilient strains.
Microbiomes and Host Evolution
The microbiome, the collection of microorganisms living in association with a host, plays a vital role in shaping the evolution of species. The interactions between a host and its microbiome can influence various physiological processes, from metabolism to immune responses.
Mutualistic Relationships: Many organisms have co-evolved with their microbiomes. For example, the human gut microbiome is essential for digestion, vitamin production, and immune system regulation. In insects, such as termites, symbiotic bacteria enable the digestion of cellulose. These mutualistic relationships can drive the evolution of both the host and the microorganisms, leading to the development of new traits that optimize this symbiosis.
Coevolution and Speciation: The tight relationship between hosts and their microbiomes can lead to coevolution, where changes in one species drive adaptations in the other. Over time, these interactions can result in speciation, where new species arise due to evolutionary pressures to maintain beneficial host-microbiome relationships.
Pathogens and Host Defense Evolution
Pathogens, including bacteria, fungi, viruses, and protozoa, exert strong selective pressures on their hosts, driving the evolution of defense mechanisms. Arms Race Dynamics: The relationship between pathogens and their hosts often results in an evolutionary “arms race.” Pathogens evolve to evade the host’s immune system, while hosts simultaneously evolve new defense mechanisms to protect against infections. This constant cycle of adaptation can lead to significant evolutionary changes in both species.
Genetic Adaptation: For instance, the evolution of the vertebrate adaptive immune system, with its capacity for vast antibody diversity, is partially a result of continuous interactions with a wide range of pathogens. Similarly, genetic mutations that confer resistance to specific pathogens (like the CCR5-Δ32 mutation, which provides resistance to HIV) are often favoured in populations exposed to those pathogens.
Microbial Influence on Gene Regulation and Epigenetics
Microorganisms can impact the regulation of host genes and epigenetic mechanisms, which are processes that affect gene expression without altering the underlying DNA sequence.
Epigenetic Modifications: Microbes can induce epigenetic changes in their hosts. For example, certain bacteria can modify histones (proteins around which DNA is wrapped) or alter DNA methylation patterns, leading to changes in gene expression. These changes can affect various traits, such as immune responses, metabolism, or even behaviour. Some of these epigenetic modifications can be inherited by subsequent generations, influencing evolutionary trajectories.
Gene Regulation and Expression: Microbial metabolites (like short-chain fatty acids produced by gut bacteria) can influence host gene expression, affecting various physiological functions. Over time, the consistent influence of these microbial products can lead to selective pressures that shape the host’s genetic architecture, favouring traits that maintain a beneficial microbiome.
Microbial Influence on Ecosystems and Niche Construction
Microbes play a significant role in shaping ecosystems and altering the environmental conditions in which species live, thereby influencing evolutionary pressures and species evolution.
Ecosystem Engineers: Certain microorganisms, such as cyanobacteria, can produce oxygen through photosynthesis, drastically changing the composition of Earth’s atmosphere and enabling the evolution of aerobic life forms. Similarly, nitrogen-fixing bacteria convert atmospheric nitrogen into forms usable by plants, influencing plant growth and, consequently, the evolution of herbivores and their predators.
Niche Construction: Microbes can also modify their own environments, creating new niches for themselves and other organisms. For example, microbial communities in soil can affect nutrient cycling, which in turn influences plant evolution. As microbes adapt to changing conditions, they can alter the evolutionary pressures experienced by other species in the same environment
Microbial Symbiosis and Adaptation
Symbiotic relationships between microbes and other species can lead to significant evolutionary adaptations.
Endosymbiosis: The theory of endosymbiosis suggests that organelles like mitochondria and chloroplasts originated from free-living bacteria that formed symbiotic relationships with ancestral eukaryotic cells. This relationship was so beneficial that these bacteria became permanent, integral components of eukaryotic cells, fundamentally altering their biology and enabling the evolution of complex multicellular organisms.
Mutualistic and Commensal Relationships: Symbiotic relationships such as those between ruminants (like cows) and their gut microbes allow for the digestion of cellulose, a plant component indigestible without microbial assistance. This mutualistic relationship has allowed ruminants to exploit niches that would otherwise be unavailable, driving their evolution into various species adapted to grazing.
Microbial Drivers of Speciation Events
Microbes can directly drive speciation events by influencing reproductive isolation and hybridization.
Reproductive Isolation: In some cases, microbes can influence the reproductive compatibility of their hosts. For instance, Wolbachia bacteria infect many insect species and can induce cytoplasmic incompatibility, where infected males can only successfully reproduce with females carrying the same strain of Wolbachia. This can lead to reproductive isolation and potentially contribute to speciation.
Hybridization and Introgression: Microbes can also affect the hybridization of species. For example, specific viruses or bacteria may only infect certain host species, thereby reducing hybrid viability or fertility when two different species interbreed. Such microbial influences can reinforce species boundaries, promoting speciation.
Microbial Influence on Behavioural Evolution
Microorganisms can impact host behaviour, thereby influencing evolutionary fitness and adaptive traits.
Behavioural Manipulation: Some parasites manipulate the behaviour of their hosts to increase their transmission. For example, the protozoan Toxoplasma gondii can infect rodents and alter their behaviour, making them less fearful of predators, thereby increasing the chances that the parasite will reach its definitive host (typically a cat). Such behaviour-altering parasites can drive the evolution of host defenses and counter-adaptations.
Microbiome and Social Behaviour: Studies have shown that the composition of the gut microbiome can influence social behaviour in animals, including humans. Changes in microbial communities can affect mood, anxiety, and social interactions, which may lead to evolutionary changes in behaviour and social structures.
Microbiology plays a crucial role in genome remodelling, with bacteria emerging as the clear winner and a significant factor in driving positive aspects of human and species evolution. Bacteria, due to their rapid mutation rates, vast genetic diversity, and ability to exchange genes across taxa, serve as powerful agents of adaptation. These mechanisms enable them to confer beneficial traits such as enhanced immunity, resilience, and even potential cognitive advantages to their hosts. While fungi and protozoa also contribute to evolutionary dynamics, bacteria’s unparalleled adaptability and influence on genetic modification highlight their primary role in shaping evolutionary outcomes. Understanding these microbial interactions further deepens our insights into evolutionary processes and offers promising pathways for advancing human health, ecology, and biotechnology.